Transequatorial Radio Propagation
Contents
- Introduction
- Transequatorial Propagation
- The Equatorial Ionosphere
- aTEP (Afternoon TEP)
- eTEP (Evening TEP)
- Diagnostics for TEP
- Australian Beacons Suitable for TEP Investigations
- Further information
Introduction
Most ionospheric models consider the ionosphere as a series of horizontal layers that vary only slowly with time and geographical location. Propagation modes that are based on such a model are called normal propagation modes. However, the real ionosphere does not always conform to this simple model, particularly in the equatorial and polar regions. Anomalies that exist in these region give rise to what are called 'unusual propagation modes'. Features of the ionosphere that give rise to these unusual modes include sporadic-E, the equatorial ionisation enhancements, ionospheric tilts at twilight, and ionospheric irregularities such as equatorial spread-F.
Two main features of the equatorial ionosphere give rise to the phenomena known as transequatorial propagation or TEP.
Transequatorial Propagation
Military and amateur radio operators in the 1940's may have been the first to discover that it was possible to communicate from north to south and vice versa across the equator over intercontinental distances using frequencies in the VHF band (QST, October 1947). At times of high sunspot number, the F2 layer may support normal modes up to 45 MHz, but frequencies considerably higher than this were found useable on transequatorial circuits. Although use was made of this phenomenon, it was not until several decades later that the actual mode of propagation was determined.
Radio amateurs soon recognised TEP as a mode worth working. The first large scale TEP communications probably occurred around 1957-1958 during the peak of solar cycle 19. Around 1970, the peak of cycle 20, many TEP contacts were made between Australian and Japanese radio amateurs. With the rise of cycle 21 starting around 1977, amateur contacts were made between Greece/Italy and Southern Africa (both South Africa and Rhodesia/Zimbabwe), and between Central and South America by TEP.
It was observed that there were two distinctly different types of TEP that could occur: The first type occurred during the late afternoon and early evening hours and was generally limited to distances under 6000 km. Signals propagated by this mode were limited to the low VHF band (<60 MHz), were of high signal strength and suffered moderate distortion (due to multipath). Single sideband voice communications were possible with this mode.
The second type of TEP occurred from around 1900 to 2300 hours local time. Contacts were made at 144 MHz, and even very rarely on 432 MHz.
The signal strength was moderately high, but subject to intense rapid fading, making morse code (narrow band CW) the only possible communication mode. One amateur described the signal quality in the following words: "we tried SSB but there was so much distortion that not a single word could be identified. [this mode] has a lot of flutter and fading and ... even the morse comes through like a breathing noise, not a clear tone" (from the Dawn of Amateur Radio in the UK and Greece by Norman F Joly).
The Equatorial Ionosphere
For convenience, the ionosphere can be divided into three zones for the purposes of characterising its behaviour: the equatorial zone, the temperate zone and the polar zone. The temperate ionosphere is, as its name implies, the "best behaved". It is also the best studied, because most of the technological societies of the world are located in this area (at least in the northern hemisphere).
Compared to the temperate zone, the Sun is more directly overhead in the equatorial zone, and so we should expect to find higher ionospheric critical frequencies than in the temperate zone. We might also expect to find a smaller variation of the ionosphere with the seasons (since essentially the tropics do not have a well defined summer and winter). At the other extreme, we would expect the polar ionosphere to be less dense (because of the high solar zenith angle), and to show the greatest variability between summer and winter. Although these expectations are essentially true, they do not explain the many interesting features of these regions. And the most important layer of the ionosphere, the F region, does not always obey these reasonable assumptions.
The polar and equatorial ionospheres are both subject to a wider range of normal and unexpected behaviour. The high latitude ionosphere was subjected to intensive study before and during the International Geophysical Year (IGY 1957-8). One of the main causes of the unexpected behaviour is the Earth's magnetic field. In the polar regions, the magnetic field lines are almost perpendicular to the Earth’s surface, while in the equatorial region, the magnetic field lines are horizontal to the Earth’s surface at the magnetic equator. (Note: to confuse the picture even further, the geomagnetic and geographic equators do not usually coincide, and they may be up to 12 degrees apart. At Asian longitudes the geomagnetic equator is the above the geographic equator, whereas at American longitudes it is below.)
The most interesting feature of the tropical ionosphere is the region commonly called the equatorial anomaly. Historically, this name arose because the ionisation peak was not expected – it’s presence disobeyed the simple mid-latitude model people had devised for the ionosphere. While we now know better what causes the ionisation crests, the old name still sticks. This is where a high electron concentration is observed on each side of the magnetic equator at magnetic latitudes at around 10 to 20 degrees. These crests of ionisation give rise to higher ionospheric critical frequencies (foF2) than exist at the geomagnetic equator. They are also at lower altitudes than is the peak of the F-layer at the geomagnetic equator.
The equatorial anomaly is caused by the combined action of electric and magnetic fields. When the overhead Sun creates intense ionisation in the region, the electric field starts these charges moving. The magnetic field (which only has an effect on moving charges) then causes them to drift upwards. Finally, the particles diffuse outwards, following the geomagnetic field down to where it intersects the normal F-layer This process starts immediately after sunrise and by mid afternoon the buildup in ionisation is clearly present and persists until after sunset, when no more ionisation is produced by the Sun.
During the early evening hours, while the enhanced ionisation is decreasing, vast ionisation irregularity regions can be formed by dynamic processes. It is generally thought that an instability starts low in the ionosphere, grows and propagates upward. These irregularities are blown around by ionospheric winds, breakup, and by the morning hours (0300 LT), have mostly disappeared. Generally, ionisation irregularities can be seen on an ionosonde as a thickening or spreading of the F2 layer trace. This is referred to as range or frequency spreading (depending on the mechanism involved - and it sometimes difficult to separate the two). All spreading is believed to be due to ionisation irregularities in the ionosphere. These irregularities, which (at least in the equatorial ionosphere) occur only at night-time, usually start to develop in the evening hours as a disturbance at the bottom of the ionosphere and then grow upward. They may be in the form of expanding plumes, and/or as small scale bubbles or pockets. They are aligned with the geomagnetic field lines (and are thus often referred to as field aligned irregularities FAI). These plumes, tubes, bubbles or pockets form holes, or biteouts in the local ionisation and radio waves are refracted by these discontinuities in the ionosphere. Not only do these irregularities affect HF radio propagation but they can also cause scintillations on L-band (low microwave) satellite to ground transionospheric signals.
The equatorial anomaly and the irregularities are used to explain transequatorial propagation.
aTEP (Afternoon TEP)
Afternoon transequatorial propagation is believed to by a super F mode (designated FF), in which the signal from the transmitter is first reflected by the concentration of ionisation at one of the equatorial anomaly crests to the second crest in the opposite hemisphere. From there it is reflected down to the ground receiving station. It thus suffers no ground reflection (as would be the case in the normal 2F mode), and it also passes through the absorptive D-layer only twice (instead of 4 times for the 2F mode).
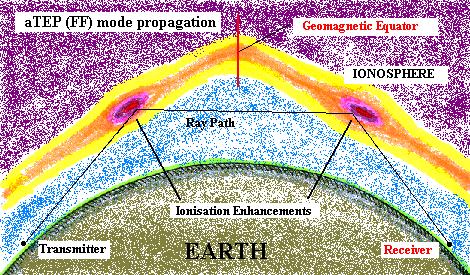
Because the intermediate ray is between two parts of the F layer, the grazing angle at the ionosphere can be substantially smaller than for a ray reflected back to the ground. This in turn implies that a higher frequency may be reflected (fr= foF2*sec(i)). Here i is the angle of incidence at the ionosphere, and as this approaches 90 (the grazing angle g=90-i tends to zero), the maximum possible reflected frequency (fr) becomes larger. Another way of saying this is that the obliquity factor of the circuit is higher. The smaller grazing angle is also made possible because the increased ionisation at the anomaly crest follows the magnetic field lines and is tilted slightly upward toward the equator.
High signal strength observed for afternoon TEP are due to the smaller number of passages through the D-layer, and because the anomaly crests support propagation of signals from a wider range of elevation angles than with usual propagation modes, the distribution of ionisation in the equatorial anomaly tending to focus these along the path.
The characteristics of aTEP are:
- Maximum useable frequency (MUF) up to about 60 MHz, which is usually about 15 to 25 MHz above the 2F mode frequency for the same path.
- Occurs from around 1500 to 1900 local time. It is more prevalent near the equinoxes and at times of high sunspot number.
- Typical path lengths will be from 5000 to 6500 kilometres.
- Signals will normally be strong with limited fading and distortion (from multipathing or Doppler spread).
eTEP (Evening TEP)
Evening transequatorial propagation generally supports much higher frequencies than aTEP and has, on rare occasions, been reported on the 432 MHz amateur frequency band (low UHF). Evening TEP is strongly correlated with the existence of range spreading, called equatorial spread F, seen on equatorial ionograms. Evening TEP propagation is not as well understood as aTEP but it is believed to take place via a whispering gallery or field-guided mode which relies on the existence of ionospheric bubbles, tubes or plumes that have an electron concentration lower than the surrounding area. Rays are reflected from the surfaces of the bubble walls, at all times staying within the ionosphere until they finally emerge on a path down to the ground.
The characteristics of evening TEP are:
- Occurs around 2000 to 2300 local time, and is more frequent around the equinoxes and especially at times of high sunspot number.
- Although signal strengths are high, the signal is subject to deep and rapid fading and very strong distortion (from multipathing and large Doppler motions). Doppler spread up to 2kHz has been observed on a CW signal.
- Path lengths may vary from 3000 to 8000 kilometres.
- Frequencies supported are higher than for aTEP and may very occasionally rise into the low UHF band.
Diagnostics for TEP
It is not yet possible to predict the occurrence of TEP with any certainty, so further investigations into these propagation circumstances are required.
We know some necessary conditions for TEP, but we also know that these are not sufficient to ensure that TEP will occur. Some of these are:
- For the highest frequency support, the circuit should be symmetric with respect to the geomagnetic equator. That is, the receiver and transmitter should be located at equal distances from the magnetic (dip) equator.
- The path must be within about 15 degrees of geomagnetic north-south.
- The occurrence rate is greatest around the equinoxes.
- Occurrence rate is greatest at times around the maximum of the solar cycle. This is a time of higher solar EUV output (which leads to more intense ionospheric ionisation).
- Occurrence rate decreases as the circuit frequency increases.
- Range spreading on equatorial ionograms appears to be a necessary but not a sufficient condition for eTEP. However, this probably depends on thestation location.
- The higher the F2 layer over the geomagnetic equator the higher the occurrence rate of TEP. In fact, this is thought to be one of the best predictors for eTEP.
- The further the equatorial anomaly crests are from the geomagnetic equator the higher the probability that aTEP will be present. This geometry favours enhancement of the FF mode.
- Quiet geomagnetic conditions appear to favour the development of ionospheric irregularities, and thus eTEP. A 27-day periodicity has been noted on some paths (probably related to geomagnetic activity of solar origin). The higher the circuit frequency, the more important it appears to be to have quiet geomagnetic conditions. (Note: geomagnetic disturbances are usually more prevalent and more intense around the equinoxes, and thus we have two conflicting conditions).
Note that some of the above diagnostics are for aTEP but many relate to eTEP.
Australian Beacons Suitable for TEP Investigations
The ideal beacon for TEP investigations is a continuous wave (CW) transmitter. This allows measurements of signal strength, Doppler shift and spread, to be made without any confounding factors introduced by the signal modulation. Failing a CW beacon, an AM transmitter is the next best substitute, as the carrier is a fixed frequency and relatively constant in power (although the total radiated power may be constant, the ratio of energy spread across carrier and sidebands may change). An FM transmitter is normally not appropriate as a TEP beacon (although the total radiated power is constant, this is spread over a wide frequency range [eg 250 kHz], and there is no energy peak at the nominal carrier frequency when significant modulation is present).
The list of Australian beacons shown below have been chosen as potential candidates for Australia- Japan TEP investigations.
Amateur Radio Beacons
Callsign | Location | Latitude | Longitude | Frequency | Power | Direction | Modulation |
---|---|---|---|---|---|---|---|
VK8VF | Darwin | -12 | 131 | 28.268 | 25W | Omni | CW |
VK8VF | Darwin | -12 | 131 | 50.310 | 10W | Omni | CW |
Further information
Leo F McNamara, The Ionosphere: Communications, Surveillance, and Direction Finding, Kreiger (Orbit Books) 1991, ISBN 0-89464-040-2.
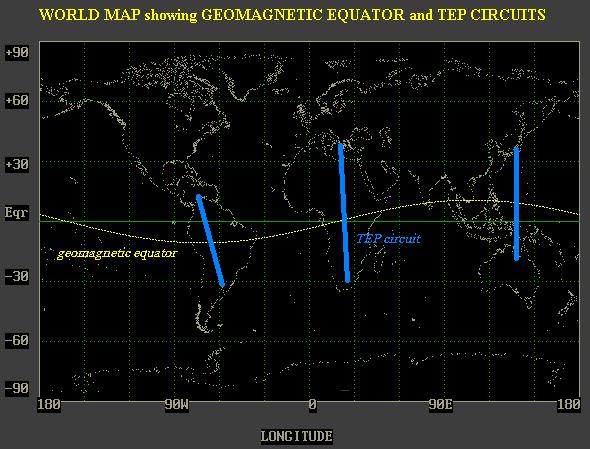